Outgoing longwave radiation
Outgoing longwave radiation (OLR) is the energy emitted by the Earth's surface and atmosphere in the form of thermal infrared radiation. Primarily within the infrared portion of the electromagnetic spectrum, outgoing long wave radiation spans wavelengths ranging from 3–100 microns (μm). It can also be referred to as upwelling longwave radiation and terrestrial longwave flux, among others. The flux of energy transported by outgoing longwave radiation is measured in watts per meter squared (Wm−2). Longwave radiation includes processes of absorption, scattering, and emissions from atmospheric gases, aerosols, clouds and the surface - however OLR represents the total amount of radiation released to space.
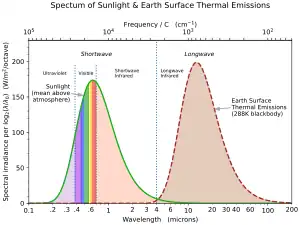

Over 99% of outgoing longwave radiation has wavelengths between 4 μm and 100 μm,[1] in the thermal infrared part of the electromagnetic spectrum. Contributions with wavelengths larger than 40 μm are small, therefore often only wavelengths up to 50 μm are considered. Specifically, earth scientists refer to the region from 8-15 μm as thermal infrared, this range is ideal for studying the longwave thermal energy that radiates from our Earth and it's atmosphere.[2] In the wavelength range between 4 μm and 10 μm the spectrum of outgoing long-wave radiation overlaps that of solar radiation, and for various applications different cut-off wavelengths between the two may be chosen.
Radiative cooling by outgoing long-wave radiation is the primary way the Earth System loses energy. The balance between this loss and the energy gained by radiative heating from incoming solar shortwave radiation determines global heating or cooling of the Earth system (Energy budget of Earth's climate).[3] Local differences between radiative heating and cooling provide the energy that drives atmospheric dynamics.
Atmospheric energy balance

As a critical component of Earth's energy budget, OLR represents the total radiation going to space emitted by the atmosphere.[4] OLR contributes to the net all-wave radiation for a surface which is equal to the sum of shortwave and long-wave down-welling radiation minus the sum of shortwave and longwave up-welling radiation.[5]
The Earth is constantly trying to obtain radiative equilibrium, where the energy in equals the energy going out. However, if there is more solar radiation absorbed than OLR the Earth will heat up. If there is more OLR than absorbed solar radiation the Earth will cool.
Due to absorption by greenhouse gases (the most important being water vapor, carbon dioxide, methane, and ozone), the atmosphere typically absorbs most of the longwave radiation emitted by the surface.[5] Assuming no cloud cover, most of the surface emissions that reach space do so through the atmospheric window. The atmospheric window occurs in the electromagnetic wavelength region between 8 and 11 μm where the atmosphere does not absorb longwave radiation (except for the ozone band between 9.6 and 9.8 μm).[5] At wavelengths where the atmosphere absorbs surface radiation, less thermal readiation reaches higher altitudes.
The OLR balance is affected by clouds and dust in the atmosphere. Clouds tend to block penetration of upwelling longwave radiation and increase cloud albedo, causing a lower flux of long-wave radiation released into the atmosphere.[6] Clouds are effective at absorbing and scattering longwave radiation and therefore hinder the amount of outgoing longwave radiation. Low clouds primarily reflect incoming solar radiation and therefore cool the earth, while high clouds trap, scatter, and re-radiate longwave radiation back towards Earth and therefore warm the planet.[7]
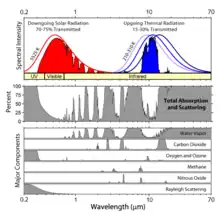
The interaction between up-welling longwave radiation and the atmosphere is complicated due to the factors that affect absorption. This absorption of longwave radiation depends on the specific absorption bands of the gases in the atmosphere.[5] The specific absorption bands are determined by their molecular structure and energy levels. Each greenhouse has a unique group of absorption bands that correspond to particular wavelengths of radiation that the gas can absorb. The path of the radiation in the atmosphere also determines radiative absorption. Longer paths through the atmosphere result in greater absorption because of the cumulative absorption by many layers of gas. Lastly, the temperature and altitude of the absorbing gas also affect its absorptivity of long wave radiation.
The net all-wave radiation balance is dominated by longwave radiation during the night and in the polar regions.[8] While there is no absorbed solar radiation, the terrestrial radiation maintains some heat at the surface.
Role in greenhouse effect
The reduction of the outgoing longwave radiative flux drives the greenhouse effect.[9] Greenhouse gases, such as methane (CH4), nitrous oxide (N2O), water vapor (H2O) and carbon dioxide (CO2), absorb certain wavelengths of OLR, preventing the thermal radiation from reaching space. This means that there is more energy entering the atmosphere than leaving, causing a warming effect. Therefore, an increase in the concentration of a greenhouse gas may contribute to global warming by increasing the amount of radiation that is absorbed and emitted by these atmospheric constituents.
If the absorptivity of the gas is high and the gas is present in a high enough concentration, the absorption bandwidth becomes saturated. In this case, there is enough gas present to completely absorb the radiated energy in the absorption bandwidth before the upper atmosphere is reached. Therefore, adding a higher concentration of this gas will have no additional effect on the energy budget of the atmosphere. However, even when a greenhouse gas is saturated at a certain wavelength, it can still absorb long wave radiation at other wavelengths within its absorption bandwidth. Other greenhouse gases may also have absorption bands that overlap with other gases leading to additional absorption of long wave radiation at those wavelengths.
The OLR is dependent on the temperature of the radiating body, see Planck's Law for more information on electromagnetic radiation emitted from a blackbody at a given temperature. It is affected by the Earth's skin temperature, skin surface emissivity, atmospheric temperature, water vapor profile, and cloud cover.[4]
OLR measurements
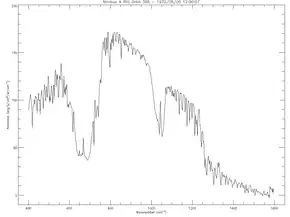
Measuring outgoing longwave radiation at the top of atmosphere and downwelling longwave radiation back towards the surface is important for understanding how much energy is kept in Earth's climate system; for example how thermal radiation cools and warms the surface, and how this energy is distributed to affect the development of clouds. Observing this radiative flux from a surface also provides a practical way of assessing surface temperatures at both local and global scales.[11]
Outgoing long-wave radiation (OLR) has been monitored and reported since 1970 by an ongoing progression of satellite missions and instruments. Earliest observations were with infrared interferometer spectrometer and radiometer (IRIS) instruments developed for the Nimbus program and deployed on Nimbus-3 and Nimbus-4.[12][13] These Michelson interferometers were designed to span wavelengths of 5-25 microns. Improved measurements were obtained starting with the Earth Radiation Balance (ERB) instruments on Nimbus-6 and Nimbus-7.[14][15] These were followed by the Earth Radiation Budget Experiment) scanners and the non scanner on NOAA-9, NOAA-10 and Earth Radiation Budget Satellite; the Clouds and the Earth's Radiant Energy System instruments aboard Aqua, Terra, Suomi-NPP and NOAA-20; and the Geostationary Earth Radiation Budget instrument (GERB) instrument on the Meteosat Second Generation (MSG) satellite.
Down-welling long-wave radiation at the surface is mainly measured by Pyrgeometer. A most notable ground-based network for monitoring surface long-wave radiation is Baseline Surface Radiation Network (BSRN), which provides crucial well-calibrated measurements for studying global dimming and brightening.[16]
OLR calculation and simulation


Many applications call for calculation of long-wave radiation quantities: the balance of global incoming shortwave to outgoing long-wave radiative flux determines the Energy budget of Earth's climate; local radiative cooling by outgoing long-wave radiation (and heating by shortwave radiation) drive the temperature and dynamics of different parts of the atmosphere. By using the radiance measured from a particular direction by an instrument, atmospheric properties (like temperature or humidity) can be inversely inferred. Calculations of these quantities solve the radiative transfer equations that describe radiation in the atmosphere. Usually the solution is done numerically by atmospheric radiative transfer codes adapted to the specific problem.
Another common approach is to estimate values using surface temperature and emissivity, then compare to satellite top-of-atmosphere radiance or brightness temperature.[8]
See also
References
- Petty, Grant W. (2006). A first course in atmospheric radiation (2. ed.). Madison, Wisc.: Sundog Publ. p. 68. ISBN 978-0972903318.
- "Infrared Waves | Science Mission Directorate". science.nasa.gov. Retrieved 2023-05-04.
- Kiehl, J. T.; Trenberth, Kevin E. (February 1997). "Earth's Annual Global Mean Energy Budget". Bulletin of the American Meteorological Society. 78 (2): 197–208. Bibcode:1997BAMS...78..197K. doi:10.1175/1520-0477(1997)078<0197:EAGMEB>2.0.CO;2.
- Susskind, Joel; Molnar, Gyula; Iredell, Lena (21 August 2011). "Contributions to Climate Research Using the AIRS Science Team Version-5 Products". NASA. Goddard Space Flight Center. hdl:2060/20110015241.
- Oke, T. R. (2002-09-11). Boundary Layer Climates. doi:10.4324/9780203407219. ISBN 9780203407219.
- Kiehl, J. T.; Trenberth, Kevin E. (1997). "Earth's Annual Global Mean Energy Budget". Bulletin of the American Meteorological Society. 78 (2): 197–208. Bibcode:1997BAMS...78..197K. doi:10.1175/1520-0477(1997)078<0197:eagmeb>2.0.co;2.
- "Clouds & Radiation Fact Sheet". earthobservatory.nasa.gov. 1999-03-01. Retrieved 2023-05-04.
- Wenhui Wang; Shunlin Liang; Augustine, J.A. (May 2009). "Estimating High Spatial Resolution Clear-Sky Land Surface Upwelling Longwave Radiation From MODIS Data". IEEE Transactions on Geoscience and Remote Sensing. 47 (5): 1559–1570. Bibcode:2009ITGRS..47.1559W. doi:10.1109/TGRS.2008.2005206. ISSN 0196-2892. S2CID 3822497.
- Schmidt, Gavin A.; Ruedy, Reto A.; Miller, Ron L.; Lacis, Andy A. (2010-10-16). "Attribution of the present-day total greenhouse effect". Journal of Geophysical Research. 115 (D20): D20106. Bibcode:2010JGRD..11520106S. doi:10.1029/2010jd014287. ISSN 0148-0227. S2CID 28195537.
- Hansel, Rudolf A.; et al. (1994). "IRIS/Nimbus-4 Level 1 Radiance Data V001". Goddard Earth Sciences Data and Information Services Center (GES DISC), Greenbelt MD USA. Retrieved 14 October 2022.
- Price, A. G.; Petzold, D. E. (February 1984). "Surface Emissivities in a Boreal Forest during Snowmelt". Arctic and Alpine Research. 16 (1): 45. doi:10.2307/1551171. ISSN 0004-0851. JSTOR 1551171.
- Hanel, Rudolf A.; Conrath, Barney J. (10 October 1970). "Thermal Emission Spectra of the Earth and Atmosphere from the Nimbus 4 Michelson Interferometer Experiment". Nature. 228 (5267): 143–145. doi:10.1038/228143a0. PMID 16058447. S2CID 4267086.
- Hanel, Rudolf A.; Conrath, Barney J.; Kunde, Virgil G.; Prabhakara, C. (20 October 1970). "The Infrared Interferometer Experiment on Nimbus 3". Journal of Geophysical Research. 75 (30): 5831–5857. doi:10.1029/jc075i030p05831. hdl:2060/19700022421.
- Jacobowitz, Herbert; Soule, Harold V.; Kyle, H. Lee; House, Frederick B. (30 June 1984). "The Earth Radiation Budget (ERB) Experiment: An overview". Journal of Geophysical Research: Atmospheres. 89 (D4): 5021–5038. doi:10.1029/JD089iD04p05021.
- Kyle, H. L.; Arking, A.; Hickey, J. R.; Ardanuy, P. E.; Jacobowitz, H.; Stowe, L. L.; Campbell, G. G.; Vonder Haar, T.; House, F. B.; Maschhoff, R.; Smith, G. L. (May 1993). "The Nimbus Earth Radiation Budget (ERB) Experiment: 1975 to 1992". Bulletin of the American Meteorological Society. 74 (5): 815–830. Bibcode:1993BAMS...74..815K. doi:10.1175/1520-0477(1993)074<0815:TNERBE>2.0.CO;2.
- Wild, Martin (27 June 2009). "Global dimming and brightening: A review". Journal of Geophysical Research. 114 (D10): D00D16. Bibcode:2009JGRD..114.0D16W. doi:10.1029/2008JD011470. S2CID 5118399.
External links
- NOAA Climate Diagnostics Center
- NASA Earth Observatory Outgoing Heat Radiation
- Office of Satellite Data Processing and Distribution, Radiation Budget at the Wayback Machine (archived May 5, 2008)
- Meteorological Satellite Center, Japan Meteorological Agency at the Wayback Machine (archived September 27, 2007)
- Planetary Energy Balance, Physical Geography